For the first time, a group of international researchers, including those from the Institute of Solid State Physics at the University of Tokyo, have demonstrated a transition, similar to a transistor, made by a single molecule called fullerene. Using carefully tuned laser pulses, researchers are able to use Fullerene to change the path of incoming electrons in a predictable way. This conversion rate can be three to six orders of magnitude larger than the conversion equipment on microchips, depending on the laser pattern used. The modification of Fullerene in networks can create computers beyond what is possible with electronic transistors, and they can lead to an unprecedented level of resolution in microscopic imaging devices.
More than 70 years ago, scientists discovered that particles transmit electrons in the direction of electricity, and later, some in the length of light. The electron emission produced a pattern that attracted attention but eluded explanation. But that has changed thanks to new, off-the-shelf research that can not only lead to advanced modern tools, but also improve our ability to explore the world itself. Project researcher Hirofumi Yanagisawa and his team discovered how electrons emitted from excited particles behave when exposed to different types of laser light, and when they tested the predictions they found out they were right.
“What we’ve managed to do here is to control how molecules conduct incoming electrons using a short pulse of red laser light,” Yanagisawa said. “Depending on the light source, the electron can stay in a default path or be moved in a predictable way. So it’s like turning on a spot on a railroad track, or an electronic transistor, only faster. We believe we can achieve switching speeds a million times faster than transistors. And that can translate to real computer performance. But one important thing is that if we can manipulate the laser to make the fullerene molecule change in many ways at the same time, it can be like having many microscopic transistors in one molecule. This can increase the complexity of the system without increasing its physical weight.
The composition of the fullerene under the switch affects perhaps the most famous carbon nanotube, although it is not a tube, the fullerene is a part of the carbon atom. When placed on a metal tip – actually the end of a pin – the fullerenes twist themselves in a certain way to direct the electrons in a predictable way. Fast laser light on the scale of femtoseconds, quadrillionths of a second, or even attoseconds, quintillionths of a second, focuses on particles that are saturated to trigger the emission of electrons. This is the first time a laser has been used to control the ejection of electrons from a molecule in this way.
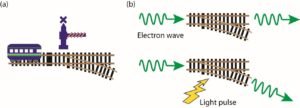
“This process is similar to how a photoelectron emission microscope produces images,” Yanagisawa said. “However, these can achieve a resolution of about 10 nanometers, or ten billionths of a meter, at best. Our full range of lights improves on this and allows a resolution of about 300 picometers, or three hundred trillionths of a meter.
In principle, since many ultrafast electronic components can be combined into a single molecule, a small amount of fullerene replacement will be enough to operate an assembly that has faster power than ordinary microchips. But there are many obstacles to overcome, such as how to make the laser part smaller, which will be necessary to create this new type of integrated circuit. So, it could be years before we see a full replacement-based phone.