By disrupting the traditional lab-based manufacturing process, Duke University researchers have greatly expanded the ability (Enhanced super-wide spectrum camera capabilities) of super surfaces to manipulate light while making them resistant to the elements. much stronger. This combination could allow these rapidly maturing devices to be used in a wide range of practical applications, such as cameras that capture wide light ranges in just a moment.
The results appear online July 1 in the journal Nano Letters.
Plasmonics is a technology that essentially traps light energy in groups of electrons vibrating together on a metal surface. This creates a small but strong electromagnetic field that interacts with the incident light. Traditionally, these groups of electrons, known as plasmons, have been excited on the surface of metal nanotubes. By controlling the size of the nanotubes and their distance from each other and the underlying metal substrate, the system can be tailored to absorb specific wavelengths of light.
This so-called plasmonic super surface consists of three layers: a metal substrate covered by a transparent nano-substrate surrounded by silver nanotubes. While this setup works well for lab demonstrations, it leaves little room for creativity. Because the nanoparticle’s area must be within a few nanometers of the underlying metal surface, the researchers couldn’t use many different shapes. To avoid this need for flatness, Maiken Mikkelsen, James N. and Elizabeth H. Barton, associate professor of electrical and computer engineering at Duke, and his team decided to try (Enhanced super-wide spectrum camera capabilities) placing each nanoparticle in its own recess or pit.
This would enclose the entire bottom half of the metal nanoparticles, allowing for the plasmon-containing faces as well as the bottoms. But because the tolerances are extremely tight, easier said than done. “We need to control certain dimensions with nanometer precision on the surface of a centimeter wafer,” says Mikkelsen. “It’s like trying to control the thickness of the blades of grass on a football field.”
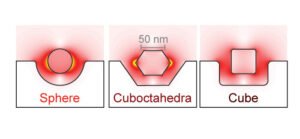
To meet this challenge, Mikkelsen and his lab fundamentally changed the traditional manufacturing process. Instead of starting with the metal surface and placing a thin transparent substrate on top, then the nanotubes, they start with the nanotubes, which are covered by a thin layer of cushioning precisely in the shape of the side. bottom and ends with a metal coating. It’s almost like an upside down pineapple cake, where the nanotubes are pineapples coated with caramelized sugar and baked into a thin bottom layer.
Because more than one surface of the nanotubes can now trap interspace plasmons, Mikkelsen and his colleagues were able to experiment in 3D with new nanoparticle shapes. In the paper, the team tested solid spheres and cubes – a figure made up of eight triangular and six square faces – as well as metal spheres with a quartz core. “Synthesis of nanoparticles can be very complex and limited for all shapes,” says Mikkelsen. “By being able to use almost any shape, we’re really opening up a lot of new possibilities, including exploring a wide variety of metals.”
The experimental results show that not only can the new fabrication method match or exceed the capabilities of previous methods using silver nanotubes, but it can also expand the frequency range exploited by different forms and these different metals. The study also found that these variations change where the nanoparticles capture energy on their surface.
Combined with the added benefit of essentially weathering the entire device by encapsulating nanoparticles, the new technique has the potential to expand the use of this technology to carry out chemical reactions or detectors. heat. However, Mikkelsen’s top priority was to apply fabrication techniques to his project to create a “super camera” capable of capturing and processing a wide range of light properties, such as polarity, depth, phase, coherence and angle of incidence.
“What’s really important here is that large macroscopic areas can be covered by super-cost surfaces very cheaply, because we’re using completely fabricated techniques,” said Mikkelsen. Do not use lithography. “This means that super surfaces can be integrated with other existing technologies and also provide inspiration for new plasmonic super surface applications.”