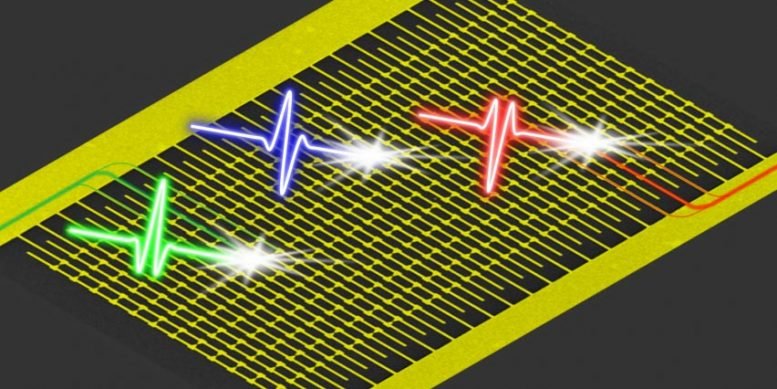
MIT researchers develop integrated lightwave electronic circuits to detect the phase of ultrafast optical fields.
Light waves oscillate far faster than most sensors can respond. A solar cell, or the infrared photodetector used to receive the signal from the remote in your DVR, can only sense the total energy delivered by the light — it can’t pick up the subtle details of the rapidly oscillating electric field the light consists of. Essentially all commercial light sensors suffer from this same problem: They act like a microphone that can tell that a crowd of people are yelling (or whispering), but can’t make out any of the individual words.
However, over the past few years, scientists and engineers have been devising clever techniques to sense the light field itself, not just the total energy it delivers. This is difficult because the required timing precision is so short — just a few femtoseconds (a femtosecond is a millionth of a billionth of a second). As a result, the apparatus and expense required for these techniques is huge, and so this work has been limited to a few specialized research laboratories. What is needed to permit wider application of this capability is an approach that is compact, manufacturable, and easy to use.
In a recent publication in the journal Nature Communications, MIT Research Laboratory of Electronics postdoc Yujia Yang and his collaborators at MIT, the University of California at Davis, the Deutsches Elektronen-Synchrotron (DESY), and the University of Hamburg in Germany have demonstrated a microchip with nanometer-length-scale circuit elements that act like antennas to collect the electric field of light oscillating at nearly 1 quadrillion times per second. The chip is small, self-contained, and requires only inexpensive electronics for readout.
Their work has the potential to enable new applications in “lightwave electronics” for high-speed signal processing using the electric field waveforms of few-cycle optical pulses. “We see a wide range of new optical and electronic devices that could be based on this technology,” says Karl Berggren, MIT professor of electrical engineering and co-author of the work. “For example, this technique could have future impact on applications such as determining the distance to remote astronomical objects, optical clocks critical to GPS technology, and chemical analysis of gases.”
To demonstrate operation of the device, the researchers first generated optical pulses using a specialized laser system, designed to make light pulses consisting of just a few optical cycles. They shined the light onto a microchip on which they had fabricated hundreds of tiny antennas patterned out of an ultrathin gold film. To get a strong enough electrical signal, the antennas had to have small gaps between them, each gap only 10 billionths of a meter wide. When the light passed through these narrow gaps, it created huge electric fields that ripped electrons out of one antenna, pulled them through the air, and deposited them on the next antenna. While each antenna on its own contributed only a tiny electrical current, the total signal across the array was substantial, and could easily be measured.