The relationship between the nanoscale structures and the way all the cells assemble and expand during this process is important. A new way to see details smaller than half the wavelength of light has revealed how nanoscale scaffolding inside cells connects to the macroscale during cell division. Unlike the previous supersolution method, the new type of supersolution monitors cell division developed and tested at the University of Michigan does not rely on particles that are boring to use for a long time.
Supersolution can reveal structures down to 10 nanometers, which are as wide as 100 atoms. He opened a new world in biology, and the first steps made it possible to win the Nobel Prize in 2014. However, its weakness is that it can take pictures in 10 minutes. This makes it impossible to see changes in cell mechanics over a long period of time.
“We were thinking – when the whole system is divided, how do the nanoscale systems interact with their nanoscale neighbors, and how does this interaction spread throughout the cell?” said Somin Lee, assistant professor of electrical and computer science at UM, who led the study published in Nature Communications.
To answer this question, Lee and his colleagues needed a new type of conclusion. Using their new method, they can monitor a single cell for up to 250 hours.
“A living cell is a busy place with proteins moving here and there. Our comfort is very good for visualizing these difficult programs, “Guangjie Cui, Ph.D. student in electrical and computer engineering and first author of the paper and Yunbo Liu, Ph.D. graduate in electrical and computer engineering.
Like the first method, the new method uses probes close to the nanoscale objects of interest and illuminates them. Superresolution 1.0 uses fluorophores, molecules that will emit light in response when processed. If the fluorophores are closer than the size of whatever is being imaged, the image can be reconstructed from the bursts of light produced by the fluorophores.
The new technique uses gold nanorods, which don’t break from repeated exposure to light, but are more resistant to light. Nanorods respond to the timing of the light, or where it is in the up and down oscillations of the electric and magnetic fields that make it. This interaction depends on how the nanorod is oriented with respect to the incoming light.
Like fluorophores, nanorods can attach to certain types of cells with targeted molecules on them. In this case, the nanorods need actin, a protein that organizes smooth cells. Actin is like branched filaments, each about 7 nanometers (about one millionth of a millimeter) in diameter, although they are joined together for thousands of nanometers.
Although nanorods are usually more than twice the size of actin, the data they provide as a group can shed light on its meaning.
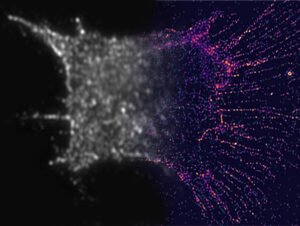
To find the nanorods, the team built filters with tiny polymers and water crystals. These filters helped to detect light at the same time, allowing the team to select nanorods with certain dimensions and incoming light.
By taking 10 to 30 images – each looking at a different area of the nanorods – and combining them into a single image, the team was able to describe the nanoscale details of the filaments inside the cells. These details will be broken down into common microscopes.
Using this method, the team discovered three rules that govern how actin organizes itself during cell division:
Actin spreads to its neighbors when actin filaments are moved. Actin will go to its neighbors to increase the connection, although this behavior is provoked by the drive to expand and reach the neighbors.
As a result, the actin network contracts when it is connected, and expands when it is not connected. The behavior of the actin is connected to the behavior of the cell—but the cell contracts when the actin expands, and it expands when the actin contracts.
The team wants to explore this further, discovering why the motions are opposite at different scales. They also want to study the consequences of a deregulation of this molecular process: is this the cause of certain diseases?
More broadly, they hope to use superresolution to understand how self-organization is embedded in biological structures, without the need for central control. “Our genomes don’t include enough information to map all the details of the process,” Lee said.
“We want to explore the process of collective behavior without a central coordination that is similar to a bird in flight and organization – in which the process is guided by the relationship between each part.”
Source: University of Michigan