Graphene is the strongest material of all. On top of that, it is an excellent conductor of heat and electricity, making it one of the most unique and versatile materials we know. For all these reasons, graphene was awarded the Nobel Prize in Physics in 2010. But many properties of the material and its relatives are still not well known – because the material is simple and atomic those who draft it are strong, hold on to keep. A group of researchers at the University of Amsterdam and New York University have now found a surprising way to (We can see the Graphene Growing) solve this problem.
The two-dimensional material, which consists of a very thin layer of atomic crystals, has attracted a lot of attention recently. This well-deserved attention is due to their rarity, which is very different from their “sweet” three-dimensional counterparts. Graphene, a famous representative, and many other two-dimensional materials are the subject of intense research in the laboratory. Perhaps surprisingly, the defect, where the crystal is not good, is very important for the different properties of these things. There, the structural arrangement of the layers of atoms is disrupted and the arrangement of atoms changes locally.
Visualize Atoms
Although defects have been shown to be very important for properties and are almost always present or applied on purpose, much is not known about their formation and evolution over time. The reason for this is simple: atoms are too small and move too fast to follow them directly.
In an effort to make the dots in graphene as visible (We can see the Graphene Growing) objects, a group of researchers from the UvA-Institute of Physics at New York University has discovered a way to build a microscale model of atomic graphene. To achieve this, they used the so-called “Uneven particles”. These elements – large enough to be easily seen under a microscope, and small enough to reproduce many of the characteristics of real atoms – interact in the same structure as the atoms of graphene and form a single structure. The researchers built a model and used it to better understand spots, their formation and their evolution over time. Their findings were published in Nature Communications this week.
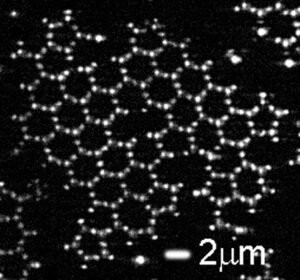
Make Graphene
Graphene is made up of carbon atoms each with three neighbors, arranged in the famous “honeycomb” pattern. It is this process that gives graphene its unique mechanical and electronic properties. To achieve the same process in their model, the researchers decorated it with a small amount of different polystyrene, with three small patches of what is known as 3-(trimethoxysilyl) propyl – or TPM. The structure of TPM patches mimics the structure of carbon fiber and graphene lattice. The researchers then modified the patches so that the particles could form bonds between them, again by analogy with the carbon atoms of graphene.
After being left alone for a few hours, when viewed under a microscope, the “fake carbon” molecules actually organized themselves into honeycomb strands. The researchers looked in detail at the defects in the graphene lattice model. They found that in this area, too, the model works: it showed the characteristic dot pattern that is also known from atomic graphene. Unlike real graphene, the direct observation and long-term structure of the model now allows scientists to find these defects from the beginning of their structure, through the intervention of the network.
Unexpected results
The new discovery and growth of graphene-like materials has led to immediate attention in both areas. Unexpectedly, the researchers found that the most common type of deformity develops already at the beginning of growth, when the network has not yet reached. They also saw how the network’s discontinuity is “corrected” by additional dots, leading to a stable pattern of dots, which stays or heals only slowly along the perfect network.
As a result, the model system not only makes it possible to reproduce the graphene network on a large scale for any kind of application, but the visualization allows a better understanding of the atomic dynamics in classes of materials. a. As the main defect and characteristic of all thin materials, these observations and model systems help to design atomic counterparts, for example for applications in ultralight devices and optical and electronic devices.
Source: University of Amsterdam