Treadmill for microswimmers provides a visual and practical look Meacham is leading the WashU team in developing acoustic microfluidic devices to study swimming microorganisms.
A team from the McKelvey School of Engineering at Washington University in St. Louis at the Massachusetts Institute of Technology has developed an acoustic microfluidic system that provides new opportunities for conducting experiments on liquid cells and microorganisms.
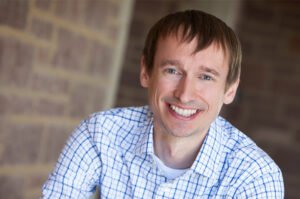
“The organisms we study are strong swimmers for their size, so the forces required to hold them are large,” J. Mark Meacham, assistant professor of engineering and materials science at the McKelvey School of Engineering and the author of the book, published June 16 in the Proceedings of the National Academy of Sciences. “In our device, ultrasonic waves like those used for imaging are able to capture the cell body without affecting the way it swims.”
The cell used in the research is the single-celled alga Chlamydomonas reinhardtii, a model used to study the movement of cilia, tiny hair-like structures that move water and move cells.
The new program was inspired by previous work in the laboratory of Philip Bayly, Professor Emeritus Lee Hunter and chair of the Department of Mechanical Engineering and Materials Science, which studies cilia movement, and Susan Dutcher, professor of genetics at the University of Washington School of Medicine, an expert on the structure and function of cilia, both co-authors.
Meacham developed the method and device with first author Mingyang Cui, who received master’s and doctoral degrees in mechanical engineering from McKelvey Engineering in 2017 and 2021, respectively, and is now a postdoctoral fellow at Massachusetts Institute of Technology.
Reinhardtii cells are tiny – and their cilia are tiny – but they swim about 10 body lengths per minute. Their eyelids also beat about 60 to 70 times per minute.
“Part of the logic and resolution required to see cilia movement is that the cells are swimming and swimming from where you’re looking very quickly,” Meacham said. “It’s hard to study their swimming behavior without trapping the cells in some way.”
Cui overcame the trap problem by using a combination of two types of waves. Surface waves create noise that travels on the surface, and large sound waves are created by vibrating the surface and water where the particles are located.
“Cells are held together by shock waves in the fluid at what are called nodes or low pressure areas,” Meacham said. “We want to use high-frequency waves because they allow for high frequencies that give small scars and less distance between them, and it gives better control over the cells you’re trying to fix.”
Unfortunately, high-efficiency wireless devices are not as efficient as their passive counterparts, and efficiency is required to generate enough trapping power in these cells to keep them from overheating.
“Not having any energy leads to heating, and that kills the cells,” Meacham said. “Mingyang has proposed a device system that uses glass microchannels, which can convert high-frequency waves into high-frequency sound waves to improve performance. The use of glass also allows us to use high-quality oil immersion microscopy.
“Having solved these practical challenges, we can focus on other benefits of acoustofluidic trapping,” said Meacham. “The important thing for our partners is to capture these cells without inhibiting their replication. The acoustic trap allows this because it does not directly enter the cells.
At first, to study the swimming cells of C. reinhardtii, the researchers used a suction pipette to hold the cells in place where the cilia were imaged. However, this does not allow the cell body to move even slightly in response to the beating of the eyelids, especially preventing the rotation of the cell, which is a natural movement when swimming.
“Think of it as a scaffold for these microswimmers, and the music field provides a way to capture the cell without affecting cilia movement or swimming in three spaces,” Meacham said.
The device also has other advantages for experimental work on microswimmers.
“We can create 25 to 30 traps at a time and do all the analysis of the trapped cells at the same time,” Meacham said. “You can’t do this with a micropipette – it’s physically impossible. That way you can make measurements quickly and with a large number of cells.
Bayly said he is excited about the implications of this work for understanding cell motility.
“Mingyang’s results show that the system does not affect swimming in any way, but the biggest effect may be on the change of the way to catch swimming cells or microorganisms of that size,” said Bayly. “You can now do many new experiments to answer unanswered biological questions by using sound traps to provide a controlled environment for the experiments to take place.”
Source: Washington University in St. Louis